New Tool Reveals What Happens When We Learn
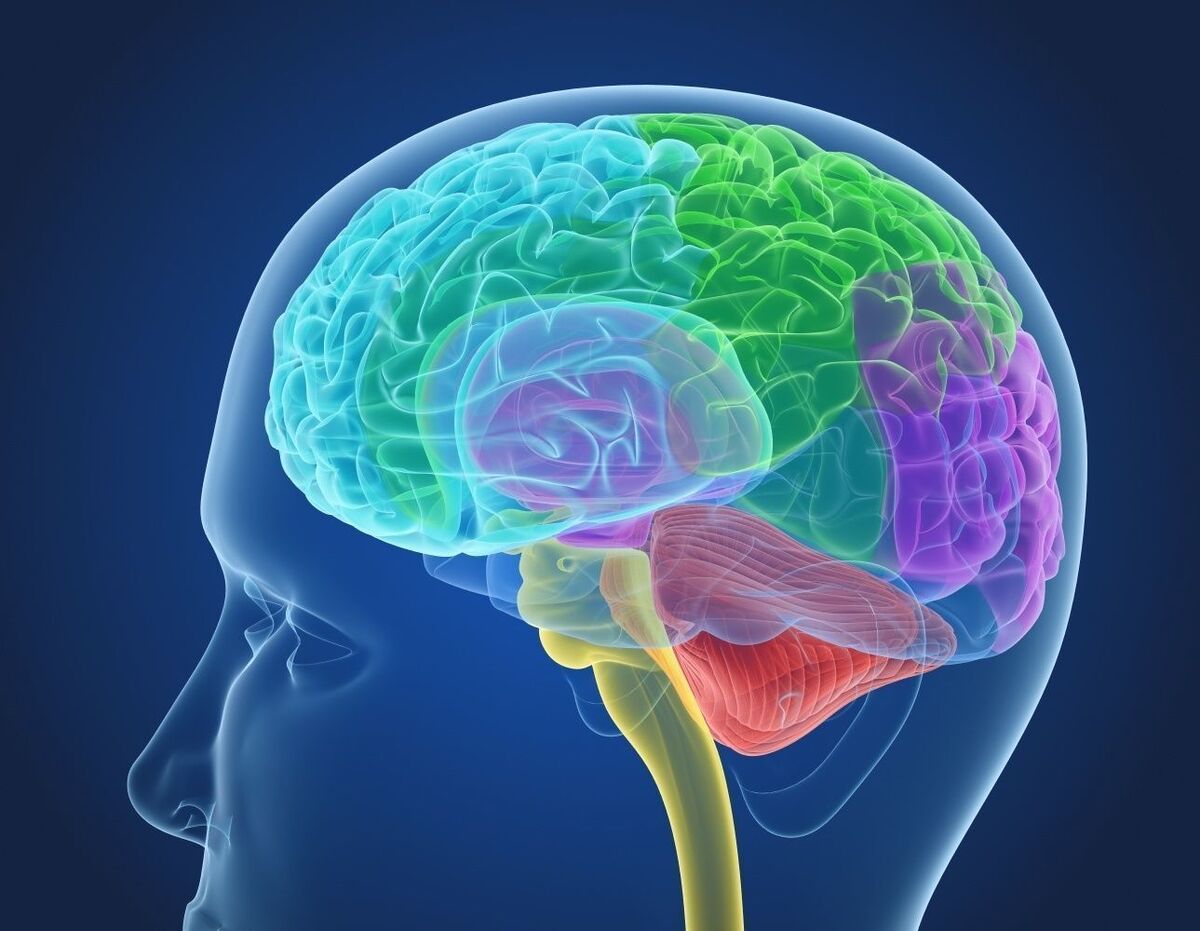
Scripps Research Institute scientists have created a new tool to monitor brain plasticity—the process by which our brains remodel and physically adjust when we learn and experience new things, such as viewing a movie or learning a new song or language. Their method, which examines the proteins generated by various brain cell types, has the potential to provide fundamental explanations for how the brain functions as well as provide insight into the many diseases of the brain where plasticity malfunctions, the Journal of Neuroscience reported.
Previous research conducted in a number of laboratories has shown how brain activity triggers changes in gene expression in neurons, an early step in plasticity. The team’s research focuses on the next important stage of plasticity—the conversion of the genetic code into proteins.
“We still don’t understand all the mechanisms underlying how cells in our brain change in response to experiences, but this approach gives us a new window into the process,” says Hollis Cline, Ph.D., the Hahn Professor and Chair of Neuroscience at Scripps Research and senior author of the new work.
Two things take place when you learn something new: First, neurons in your brain immediately transmit electrical signals along new neural pathways. This eventually results in changes to the physical structure of brain cells and their connections. But for a long time, scientists have wondered what occurs between these two steps. How does the brain eventually undergo more substantial changes as a result of this electrical activity in neurons? Also, how and why does this plasticity deteriorate with aging and certain diseases?
Previously, researchers have studied how genes in neurons turn on and off in response to brain activity, hoping to get insight into plasticity. With the advent of high-throughput gene sequencing technologies, tracking genes in this way has become relatively easy. But most of those genes encode proteins—the real workhorses of cells, the levels of which are more difficult to monitor. But Cline, in close collaboration with Scripps professor John Yates III, Ph.D., and associate professor Anton Maximov, Ph.D., wanted to look directly at how proteins in the brain change.
“We wanted to jump into the deep end of the pool and see what proteins are important to brain plasticity,” says Cline.
The team designed a system in which they could introduce a specially tagged amino acid—one of the building blocks of proteins—into one type of neuron at a time. As the cells produced new proteins, they would incorporate this amino acid, azidonorleucine, into their structures. By tracking which proteins contained the azidonorleucine over time, the researchers could monitor newly made proteins and distinguish them from pre-existing proteins.
Cline’s group used the azidonorleucine to track which proteins were made after mice experienced a large and widespread spike in brain activity, mimicking what happens at a smaller scale when we experience the world around us. The team focused on cortical glutamatergic neurons, a major class of brain cells responsible for processing sensory information.
After the increase in neural activity, the researchers discovered levels of 300 different proteins changed in the neurons. While two-thirds increased during the spike in brain activity, the synthesis of the remaining third decreased. By analyzing the roles of these so-called “candidate plasticity proteins”, Cline and her colleagues were able to gain general insight into how they might impact plasticity. Many of the proteins are related to the structure and shape of neurons, for instance, as well as how they communicate with other cells. These proteins suggested ways in which brain activity can immediately begin to impact connections between cells.
Additionally, a number of the proteins were related to how DNA is packaged inside cells; changing this packaging can change which genes a cell can access and use over a long time period. This suggests ways that a very short spike in brain activity can lead to more sustained remodeling within the brain.
“This is a clear mechanism by which a change in brain activity can lead to waves of gene expression for many days,” says Cline.
The researchers hope to use this method to discover and study additional candidate plasticity proteins, for instance those that might change in different types of brain cells after animals see a new visual stimulus. Cline says their tool also could offer insight into brain diseases and aging, through comparisons of how brain activity impacts protein production in young versus old and healthy versus diseased brains.
4155/v